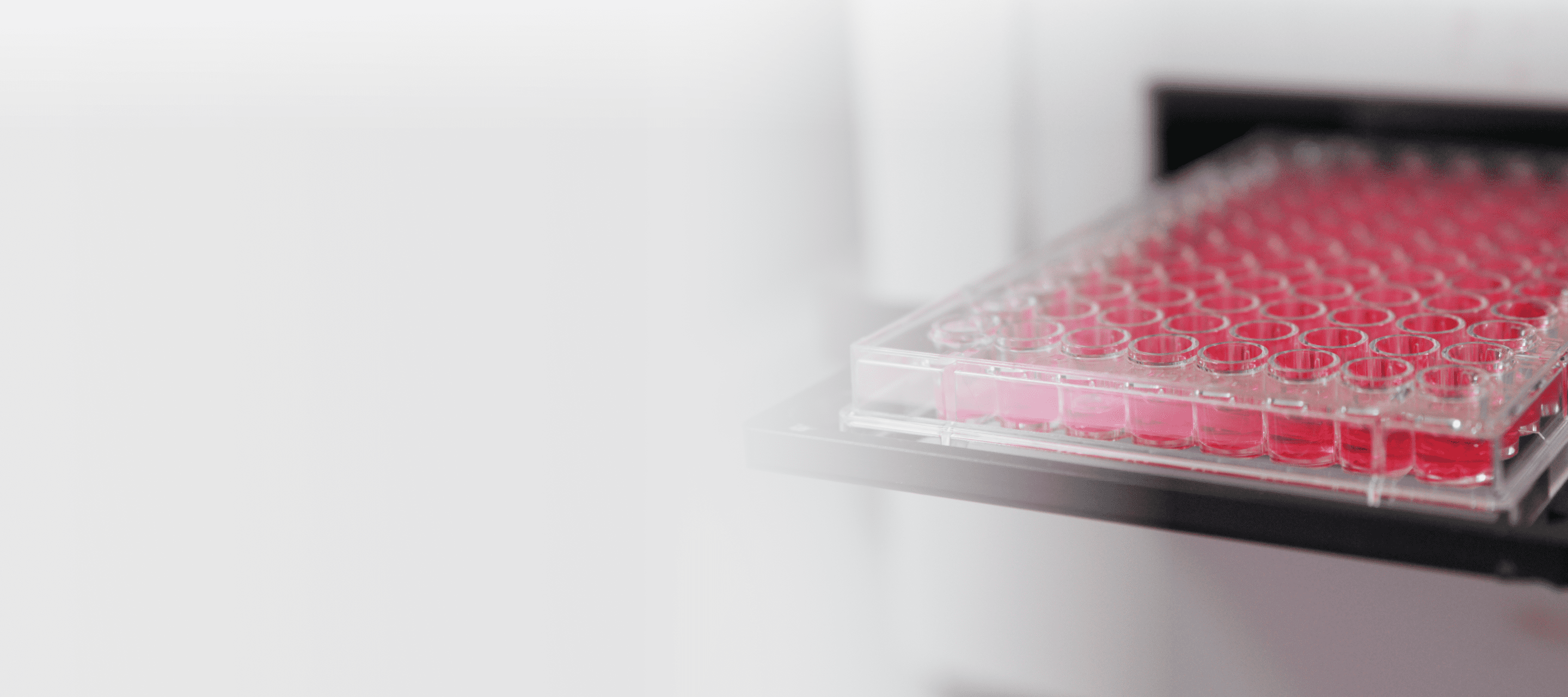
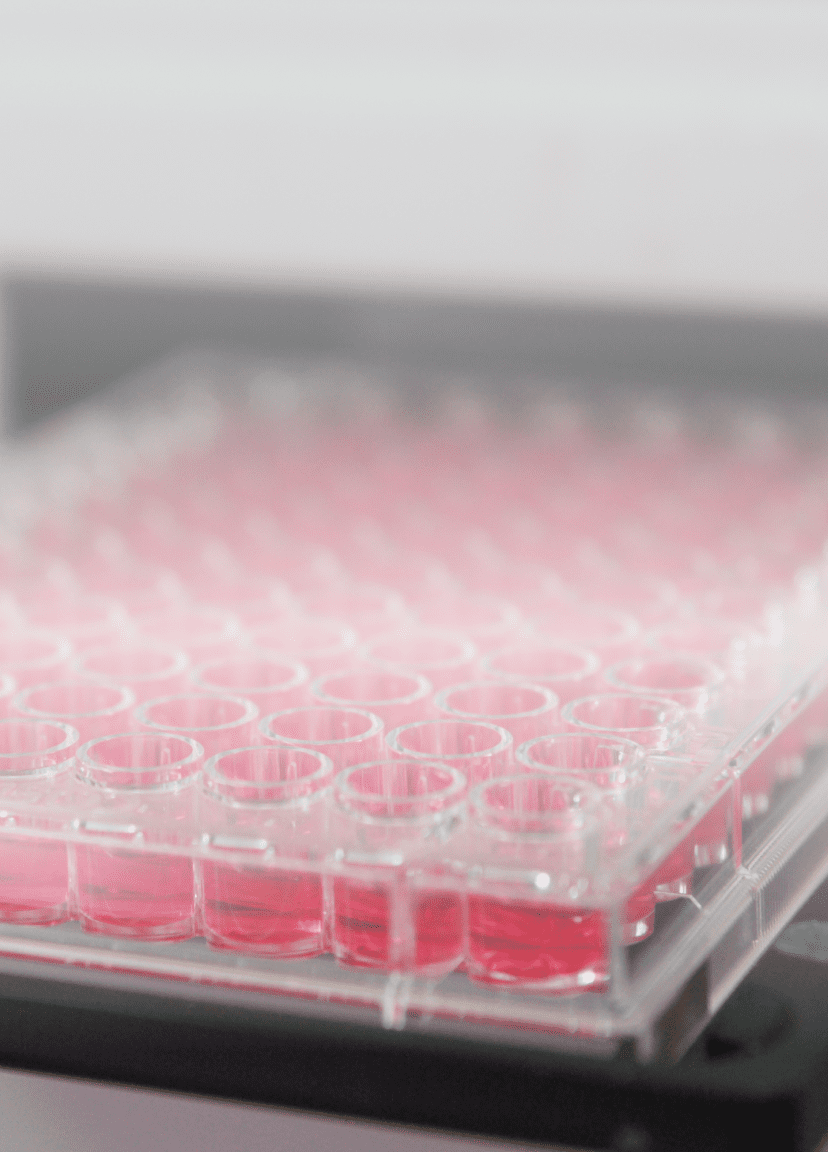
Quick automation wins in mammalian cell culture
Automating mammalian cell culture can improve the scale and quality of results for labs. We look at how labs can implement easy automation wins today
Why automate cell cultures?
Whether a lab is involved in cutting edge cancer research, drug development, cell-based vaccine development or even synthetic-food production, it needs to aim for high productivity, efficiency, and reliability to remain competitive1.
However, mammalian cell lines, as any scientist who has worked with them knows, can be rather demanding. The repetitive passaging, monitoring for health and growth, and maintenance of appropriate culture conditions can be extremely tedious, often requiring skilled scientists to be in the lab during the evening or over the weekend.
Automated cell culture systems are gaining popularity because they have the potential to overcome the drawbacks of manual cell culture by improving reliability and scalability, and by enabling the redeployment of skilled staff2–4.
Reliability
Even the most experienced and well-trained scientist can make mistakes5,6. During cell culture, this can result in abnormal growth patterns, uneven cell attachment, changes in growth rate, or contamination, resulting in experimental variability7,8,9. Automated cell culture systems are able to meet strict standardization requirements, reduce contamination rates, and minimise human error2.
Scale
If a lab can introduce automation at an early stage, while they are still managing small volumes of cell culture, they will be better able to scale up their cell processes when they are ready to do so10. Automated cell culture frameworks can be scaled up to deliver high throughput, high purity, and high yield cell growth11. They can overcome the knowledge transfer bottleneck caused by staff turnover12, perform screening on a large scale, and run cell line development projects simultaneously13.
For example, switching from a manual cell line development workflow to an automated process can mean a move from 96-well to 384-well plates, resulting in a 4-fold increase in clone density14.
Redeployment
Automated processing can replace hours of repetitive manual work with instrumentation4, meaning that skilled staff are free to perform other tasks, such as process improvements14, or research and development4.
What processes are suitable for automation?
Automation can be applied to the vast majority of cell culture processes. The methods used to achieve this can vary, but the following represent some of the approaches that have been used:
Passaging
Automated passaging can be achieved in a number of different ways. For most applications the simple combination of an automated imaging system for measurement of confluency in combination with and incubator and liquid handler will suffice. At the more specialised end a combination of an inverted microscope with a motorised stage and a robotic arm equipped to pick colonies or harvest cells by scraping may be more suited to undifferentiated PSC passaging. Images of the cell culture or the selected colonies can be stored for future reference15.
A liquid-handler and robotic arm for transposition of culture flasks and other receptacles can provide fine control over many protocol parameters, including cell culture conditions, fluid temperature, and dispensing speed3.
Cell viability, confluence, and cell counting
Automated analysis tools can provide information about cell concentration, viability, aggregation, and morphology by combining assays such as trypan blue exclusion with a high-resolution image scanner.
If the cells are ready for passaging or offline analysis, additional media can be added by a liquid-handling robot to achieve the desired cell density, and cells can be seeded by a flask- or plate-handling robotic arm.
Automated systems add the potential for a far greater number of analyses to be carried out as systems can work out of hours gathering more depth of data. For example, gathering glycan and charge variance data for greater measure of product quality in conjunction with cell health and pure product quantity data.
Media exchange and cell maintenance
One of the most tedious processes that often requires out of hours atttendence. Automated systems can be used to exchange media on a user-defined schedule, store media at cold temperatures and warm it before dispensing. This means that media exchange can be performed outside normal staff working hours, such as during the weekend.
Cryopreservation
Optimized freezing and thawing methods are required to maximise cell recovery after cryopreservation. For example, stem cells may take 2–3 weeks to be ready for further experiments after thawing and seeding, but this can be reduced to 4–7 days under optimised conditions17. Automated systems can control freezing and thawing rates to ensure consistent and viable cell lines4.
Sampling for offline analysis
Plate handling robots can work with liquid handlers to dispense accurate samples of cultured cells to 96- or 384-well plates, replace the lid of the plate, and return it to the incubator, to be retrieved later for screening or experimentation16. They can even go straight into preparation of cells for assays if required.
What makes automating cell culture challenging?
Adherent vs suspension cell culture
Many mammalian cell lines need to attach to a substrate to maintain viability and phenotype, yet automated monitoring of adherent cells poses a challenge, since the idea of a representative sample for analysis and monitoring is rarely applicable18. As a result, laboratories often convert to suspension techniques in order to increase throughput19. Suspension cells present some specific challenges before stepping into the world of bioreactors. Use of shake flasks may be need to be changed to labware that works with automated shaking incubators. Centrifuges may need to be incorporated to automated systems.
There are also tools available for mimicing the preparative steps a human would take to adherent cells. Specialised labware more suited to automation as opposed to culture flasks; tilting and knocking hardware that allows for cells to be dislodged and aspirated from corners.
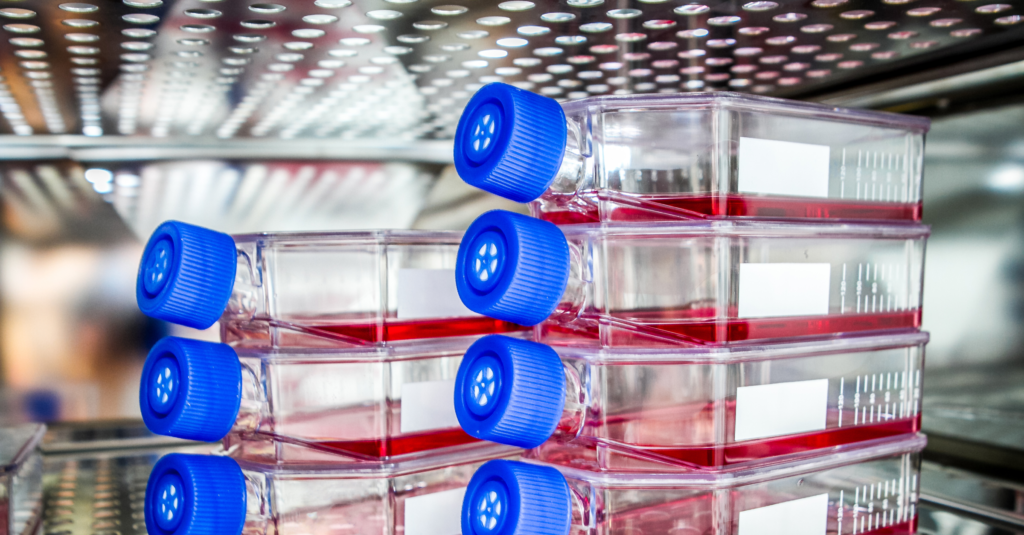
Cell culture flasks
Adherent cell culture is usually carried out in disposable plastic flasks18, which can be challenging for machinery to manipulate, requiring a robotic arm to unscrew the caps, and to tilt and knock the vessels to detach cells. To address these issues, labs need to consider using special robot-friendly culture flasks16 or microplates for their cell culture processes.
Suspension cultures, commonly grown in shake flasks, present their own automation challenges. Shake flasks can become heavy and cumbersome when large volumes of cells are required21, and automation systems need to be able to feed and sample the growing culture22. Automated incubators capable of handling suspension culture labware are very limited in capacity at this time, forcing decisions to be made about the scale of culture that is possible.
Sterility
Maintaining sterility during manual cell culture requires laminar-flow cabinets, lab coats, gloves, and careful aseptic technique. Therefore, several automated systems incorporate sterile enclosures with access to an incubator and with fresh media pumped directly into the system from a sterile storage area3,16. However, it is worth bearing in mind that automated systems may not need the same level of containment; there will be less air exchange between the cell culture environment and the rest of the lab, and less contact with (germ‑ridden) humans.
Large fluid volumes
Conventional liquid handling equipment can struggle to dispense and aspirate the large volumes of fluid that may be encountered during media exchange. Cell culture automation therefore requires specialized liquid handlers to exchange media rapidly while minimising shear force on the cells.
Data storage and processing
Automated systems need to collect huge amounts of data on multiple cell cultures, which needs to be easily accessible by laboratory scientists. This is usually achieved through the integration of a digital ecosystem including laboratory information management system (LIMS) and electronic lab notebooks (ELNs) with automated machinery and processes, offering the potential to improve workflow through machine learning and artificial intelligence23.
Automation of cell culture applications provides a unique opportunity to layer in collection of a broader dataset than would be taken with traditional manual approaches.
Traceability
Integrating automated equipment into the lab usually requires process control software often referred to as the laboratory automation system (LAS). In a cell culture system, the LAS should be to scan a cell culture’s bar code, allowing samples to be followed throughout their path through the laboratory24, so that all the data pertaining to it can be linked together in the digital ecosystem.
Summary
Automation has the potential to counter the tedium and human error involved in cell culture, freeing scientists for more cerebral activities. There is also untapped potential to gather larger datasets through automation as they can work past conventional time constrainsts and at greater scale.
By introducing more automation into cell culture processes, labs can begin to meet the rapidly increasing demands for reliability and scalability in this scientific field.
References
- Agilent Technologies. Understanding key challenges and pain points in the global laboratory market. Global Analysis. June 2017. Available at: https://www.agilent.com/content/dam/about/newsroom/infograhics/pdf/fact-sheet-lab-manager.pdf (last accessed February 2022)
- Transparencymarketresearch.com. Cell culture market – Global Industry Analysis, Size, Share, Growth, Trends, and Forecast, 2021-2028. Available at: https://www.transparencymarketresearch.com/cell-culture-market.html (last accessed April 2022)
- Kane KIW, et al. Automated microfluidic cell culture of stem cell derived dopaminergic neurons. Sci Rep 2018;9:1796
- Moore S. Cell culture automation: An overview. Available at: https://www.azolifesciences.com/article/Cell-Culture-Automation-An-Overview.aspx (last accessed 17 May 2022)
- Parliamentary Office of Science and Technology. Managing human error. Postnote 2001:156
- Leadership and Worker Engagement Forum. Leadership and worker involvement toolkit: Understanding human failure. Available at: https://www.hse.gov.uk/construction/lwit/assets/downloads/human-failure.pdf (last accessed February 2022)
- Salauddin M. A brief concept of cell culture: Challenges, prospects and application. Available at: https://www.intechopen.com/online-first/78274 (last accessed April 2022)
- Corning Inc. What’s killing my cell cultures? Troubleshooting cell growth issues. Available at: https://www.corning.com/emea/en/products/life-sciences/resources/stories/at-the-bench/what-is-killing-my-cell-cultures-troubleshooting-cell-growth-issues.html#:~:text=Generally%2C%20the%20most%20common%20issues,technique%2C%20incubation%2C%20and%20media (last accessed 17 May 2022)
- Holland I and Davies JA. Automation in the life science research laboratory. Front Bioeng Biotechnol 2020;8:571777
- Doulgkeroglou M-N, et al. Automation, monitoring, and standardization of cell product manufacturing. Front Bioeng Biotechnol 2020;8:811. https://www.ncbi.nlm.nih.gov/pmc/articles/PMC7381146/
- Report Ocean. Cell culture market new opportunities, top trends, rising demand, emerging growth, business development, and regional analysis 2030. Available at: https://www.marketwatch.com/press-release/cell-culture-market-new-opportunities-top-trends-rising-demand-emerging-growth-business-development-and-regional-analysis-2030-2022-02-14 (last accessed April 2022)
- Ochiai K, et al. A variable scheduling maintenance culture platform for mammalian cells. SLAS Technol 2021;26:209–17
- Lindgren K, et al. Automation of cell line development. Cytotechnol 2009;59:1–10
- Sargent B. Automating Cell line development workflows to improve efficiency and throughput. Cell Culture Dish 2019. Available at: https://cellculturedish.com/automating-cell-line-development-workflows-to-improve-efficiency-and-throughput/ (last accessed 17 May 2022)
- Daniszewski M, et al. Automated cell culture systems and their applications to human pluripotent stem cell studies. SLAS Technol 2018;23:315–25
- Kempner ME and Felder RA. A review of cell culture automation. JALA 2002;7(2):56-62.2002
- Uhrig M, et al. Improving cell recovery: Freezing and thawing optimization of induced pluripotent stem cells. Cells 2022;11:799.
- Jaccard N, et al. Automated and online characterization of adherent cell culture growth in a microfabricated bioreactor. J Lab Automation 2014;19:437–43
- St. Amand T and Gitschier H. Success with adherent or suspension cell cultures. Genetic Engineering & Biotechnology News 2021. Available at:https://www.genengnews.com/commentary/success-with-adherent-or-suspension-cell-cultures/ (last accessed 17 May 2022)
- Thomas R and Ratcliffe E. Automated adherent human cell culture (mesenchymal stem cells). Methods Mol Biol 2012;806:393–406
- Ward T. Automating cell culture to optimise cell line generation and selection. Drug Discovery World 2003. Available at: https://www.ddw-online.com/automating-cell-culture-to-optimise-cell-line-generation-and-selection-1354-200312/ (last accessed 17 May 2022)
- Grapov D, et al. Rise of deep learning for genomic, proteomic, and metabolomic data integration in precision medicine. OMICS 2018;22:630–636
- Hawker CD. Nonanalytic laboratory automation: A quarter century of progress. Clin Chem 2017;63:1074–1082
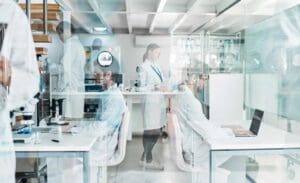
Lab layouts, until the introduction of automation, had seen little change in…
Read more Automation holds the key to unlocking the lab of the future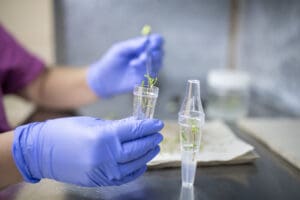
We explore common problems in plus the advantages and disadvantages of plant…
Read more Plant tissue culture: how automation can help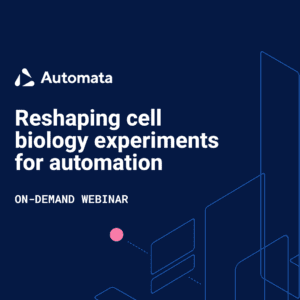
In this webinar Russell Green, Automata’s Director of Product Growth, talks about…
Read more On-demand webinar: Reshaping cell biology experiments for automation